The citric acid cycle, also known as the Krebs cycle or the tricarboxylic acid cycle, is the second stage of cellular respiration. It takes place in the mitochondria of the cell and is responsible for the complete oxidation of acetyl-CoA, a molecule derived from pyruvate, to carbon dioxide and water. This process produces ATP, NADH, and FADH2 (flavin adenine dinucleotide) as energy carriers, and it’s the main source of energy production in the cell.
The citric acid cycle is a series of enzyme-catalyzed reactions that convert acetyl-CoA into CO2 and water, releasing energy in the process. The cycle begins with the combination of acetyl-CoA and oxaloacetate to form citrate. Through a series of reactions, citrate is converted into CO2 and water, releasing energy in the form of ATP, NADH and FADH2. These energy carriers are used in the next stage of cellular respiration, the electron transport chain, to generate more ATP.
It’s worth noting that the citric acid cycle is not only a fundamental process in cellular respiration but also plays an important role in the metabolism of many organisms. It’s a central metabolic hub, where intermediates produced during the cycle are used in other metabolic pathways, such as the synthesis of amino acids and lipids.
In summary, the citric acid cycle is a metabolic process that takes place in the mitochondria of the cell, responsible for the complete oxidation of acetyl-CoA to carbon dioxide and water. It produces ATP, NADH and FADH2 as energy carriers, and it’s the main source of energy production in the cell. It’s a fundamental process in cellular respiration and also plays an important role in the metabolism of many organisms.
Table of Contents
Location of the citric acid cycle in the cell
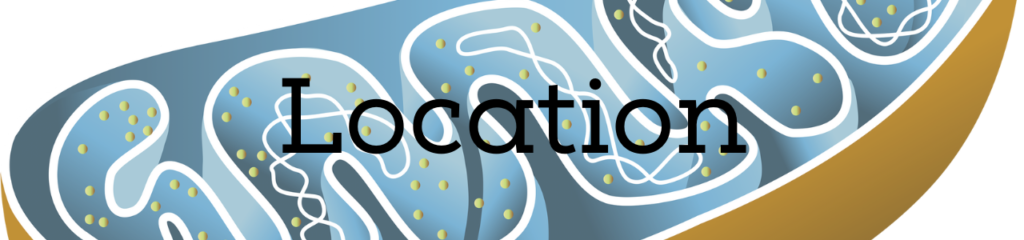
The citric acid cycle, also known as the Krebs cycle, takes place in the mitochondria of the cell. The mitochondria are organelles that are often referred to as the “powerhouses” of the cell because they generate the majority of the cell’s ATP through cellular respiration.
Mitochondria are double-membraned organelles, with an outer membrane and an inner membrane. The citric acid cycle takes place in the matrix of the mitochondria, which is the space between the inner membrane and the mitochondrial DNA. The enzymes that catalyze the reactions of the citric acid cycle are located in the mitochondrial matrix, and the cycle is also dependent on the presence of cofactors such as NAD and FAD which are also located in the mitochondrial matrix.
It’s also worth noting that the citric acid cycle is not limited to one organelle or compartment in the cell. Some of the enzymes involved in the citric acid cycle are located in the mitochondria, while others are located in the cytoplasm.
In summary, the citric acid cycle is a metabolic process that takes place in the mitochondria of the cell, specifically in the matrix of the mitochondria, where it uses enzymes to catalyze the breakdown of acetyl-CoA into carbon dioxide and water. This process generates ATP, NADH, and FADH2 as energy carriers, and it’s the main source of energy production in the cell.
Steps of the citric acid cycle process
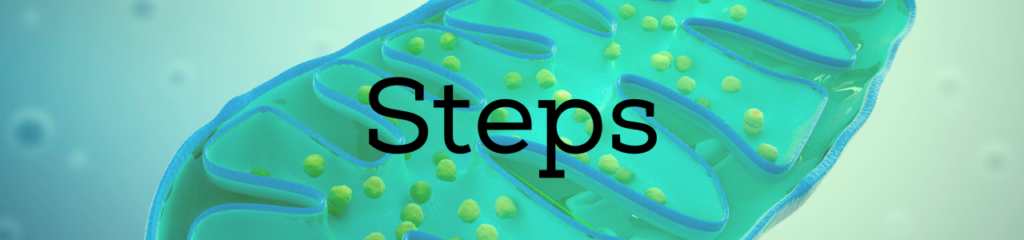
The citric acid cycle, also known as the Krebs cycle, is a series of enzyme-catalyzed reactions that convert acetyl-CoA into CO2 and water, releasing energy in the process. The cycle can be divided into the following steps:
1. Acetyl-CoA combines with oxaloacetate to form citrate, a six-carbon molecule, by the enzyme citrate synthase.
2. Citrate is then converted into isocitrate by the enzyme aconitase.
3. Isocitrate is then converted into alpha-ketoglutarate by the enzyme isocitrate dehydrogenase. This reaction also produces one molecule of NADH.
4. Alpha-ketoglutarate is then converted into succinyl-CoA by the enzyme alpha-ketoglutarate dehydrogenase. This reaction also produces one molecule of NADH.
5. Succinyl-CoA is then converted into succinate by the enzyme succinyl-CoA synthetase. This reaction also produces one molecule of GTP (guanosine triphosphate), which can be used to generate ATP.
6. Succinate is then converted into fumarate by the enzyme succinate dehydrogenase. This reaction also produces one molecule of FADH2.
7. Fumarate is then converted into malate by the enzyme fumarase.
8. Malate is then converted into oxaloacetate by the enzyme malate dehydrogenase. This reaction also produces one molecule of NADH.
9. The cycle starts again with the formation of citrate by the combination of acetyl-CoA and oxaloacetate.
In summary, the citric acid cycle is a series of enzyme-catalyzed reactions that convert acetyl-CoA into CO2 and water, releasing energy in the process. This process produces ATP, NADH, and FADH2 as energy carriers. The cycle begins with the combination of acetyl-CoA and oxaloacetate to form citrate, and ends with
the formation of oxaloacetate. The cycle then repeats itself, allowing for the continuous production of energy.
It’s worth noting that during each turn of the citric acid cycle, three molecules of NADH and one molecule of FADH2 are produced. These energy carriers are used in the next stage of cellular respiration, the electron transport chain, to generate more ATP.
It’s also important to note that the citric acid cycle is not only a source of energy production, but also plays a key role in the metabolism of many organisms. The intermediates produced during the cycle are used in other metabolic pathways, such as the synthesis of amino acids and lipids. Additionally, the cycle also helps to maintain the balance of metabolic intermediates and the balance of redox potential in the cell.
In conclusion, the citric acid cycle, also known as the Krebs cycle, is a fundamental metabolic process that takes place in the mitochondria of the cell. It converts acetyl-CoA into CO2 and water, releasing energy in the process. The cycle produces ATP, NADH, and FADH2 as energy carriers and plays a key role in the metabolism of many organisms. It’s a central metabolic hub, where intermediates produced during the cycle are used in other metabolic pathways, and it helps to maintain the balance of metabolic intermediates and the balance of redox potential in the cell.
Gross and Net Gain of ATP during Krebs Cycle
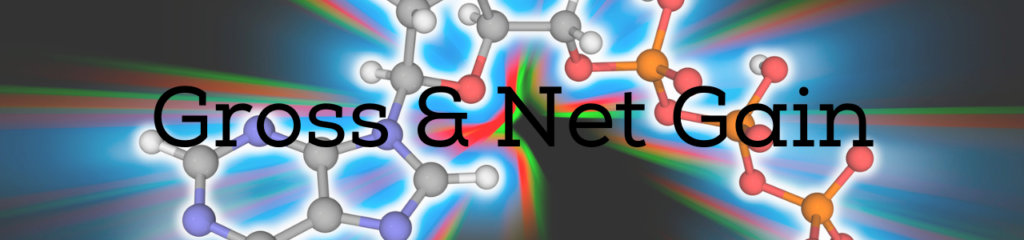
During the citric acid cycle, a single molecule of acetyl-CoA is broken down into two molecules of CO2 and water, releasing energy in the process. This process also produces ATP, NADH, and FADH2 as energy carriers.
The gross gain of ATP during the citric acid cycle is 12 ATP molecules. This is because one GTP molecule is produced during the cycle, which can be converted into ATP by the enzyme GTPase. Additionally, 3 NADH molecules and 1 FADH2 molecule are produced during the cycle, which are used in the next stage of cellular respiration, the electron transport chain, to generate more ATP.
However, the net gain of ATP during the citric acid cycle is 2 ATP molecules per acetyl-CoA molecule. This is because two ATP molecules are consumed during the energy-investment phase to convert pyruvate into acetyl-CoA and two ATP molecules are produced during the energy-payoff phase by substrate-level phosphorylation.
It’s worth noting that the net gain of ATP during the citric acid cycle can vary depending on the organism and the conditions of the cell, as the amount of ATP produced during substrate-level phosphorylation and the electron transport chain can vary. Additionally, the actual number of ATP molecules produced by the electron transport chain also depends on the ratio of NADH to FADH2 produced during the citric acid cycle, as NADH produces more ATP than FADH2.
In summary, the citric acid cycle is a metabolic process that takes place in the mitochondria of the cell and is responsible for the complete oxidation of acetyl-CoA to carbon dioxide and water. It produces ATP, NADH and FADH2 as energy carriers. The gross gain of ATP during the citric acid cycle is 12 ATP molecules, but the net gain of ATP is 2 ATP molecules per acetyl-CoA molecule. The actual number of ATP molecules produced by the electron transport chain depends on the ratio of NADH to FADH2 produced during the citric acid cycle.
It’s also important to note that the citric acid cycle is not only a source of energy production, but also plays a key role in the metabolism of many organisms. The intermediates produced during the cycle are used in other metabolic pathways, such as the synthesis of amino acids and lipids. This means that the net gain of ATP during the citric acid cycle is not only used for energy production but also for other metabolic processes.
Additionally, it’s also worth mentioning that the citric acid cycle is a highly regulated process, controlled by allosteric enzymes, hormones, and other signaling molecules. This regulation ensures that acetyl-CoA is broken down in a controlled manner, avoiding metabolic imbalances and providing the appropriate amount of energy for the cell’s needs.
The Significance of the Citric Acid Cycle
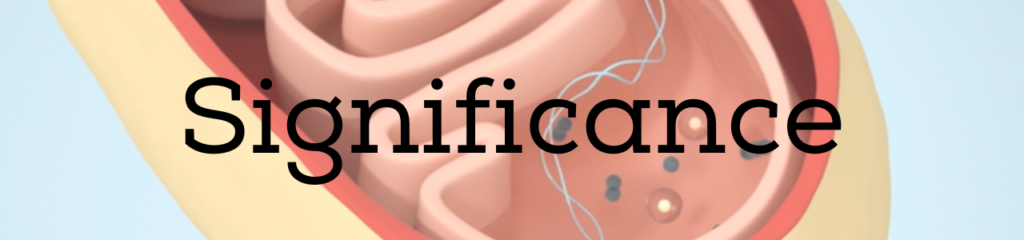
The Citric Acid Cycle is the hub of cellular respiration, connecting various metabolic pathways and supplying energy to the cell. It serves two primary functions:
A. Energy Production: Throughout the cycle, NADH and FADH2 are generated, which carry high-energy electrons. These electron carriers feed into the ETC, a series of protein complexes embedded in the inner mitochondrial membrane, driving the synthesis of ATP through oxidative phosphorylation. This process is the main source of cellular energy.
B. Biosynthesis: Apart from energy production, the Citric Acid Cycle produces intermediate molecules that are crucial for biosynthetic pathways. These intermediates contribute to the synthesis of amino acids, fatty acids, and other vital molecules essential for cellular functions.
Regulation of the Citric Acid Cycle
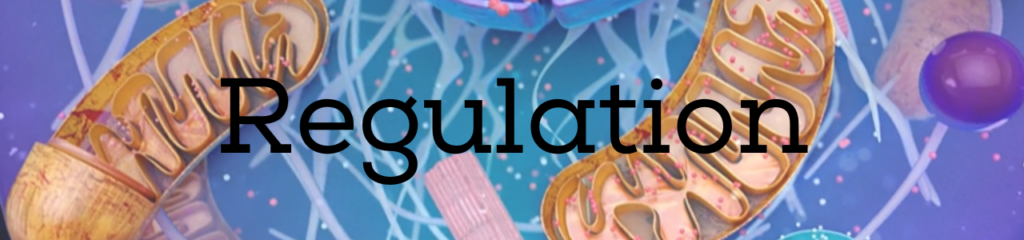
The Citric Acid Cycle is tightly regulated to maintain cellular homeostasis. The key regulatory points are the enzymes involved in the irreversible steps, such as citrate synthase, isocitrate dehydrogenase, and α-ketoglutarate dehydrogenase. The availability of substrates, ATP, and NADH also influences the rate of the cycle.
Overall, the citric acid cycle is a fundamental metabolic process that takes place in the mitochondria of the cell. It’s responsible for the complete oxidation of acetyl-CoA to carbon dioxide and water, releasing energy in the process. The cycle produces ATP, NADH, and FADH2 as energy carriers and plays a key role in the metabolism of many organisms. The intermediates produced during the cycle are used in other metabolic pathways, such as the synthesis of amino acids and lipids. The citric acid cycle is a highly regulated process, controlled by allosteric enzymes, hormones, and other signaling molecules. The net gain of ATP during the citric acid cycle is 2 ATP molecules per acetyl-CoA molecule, which is used for energy production and other metabolic processes.